Effect of tissue factor pathway inhibitor on the pyroptosis of vascular smooth muscle cells induced by angiotensin II
Highlight box
Key findings
• This study found that angiotensin II (Ang II) could promote pyroptosis in vascular smooth muscle cells (VSMCs) by activating the NOD-like receptor family pyrin domain containing 3 (NLRP3) inflammasome. Furthermore, recombinant tissue factor pathway inhibitor (rTFPI) could inhibit Ang II-induced VSMC pyroptosisby inhibiting the NLRP3 inflammasome.
What is known and what is new?
• Ang II could induce hypertension by promoting pyroptosis.
• The pathway by which rTFPI inhibits pyroptosis is unknown.
What is the implication, and what should change now?
• rTFPI play an important role in the treatment of vascular remodeling induced by hypertension.
Introduction
The hormonal mechanism activated by the renin-angiotensin-aldosterone system (RAAS) is one of the main pathogenic pathways of hypertension (1). The RAAS can regulate fluid metabolism and endothelial dysfunction as well as induce the proliferation and phenotypic transformation of vascular smooth muscle cells (VSMCs), ultimately leading to vascular remodeling (2). VSMCs are a major cell type in arterial blood vessels. When blood vessels are injured or stimulated by inflammation, VSMCs can transform from a contractile phenotype to a synthetic, proliferative, and migratory state (3). As the main effector of the RAAS, angiotensin II (Ang II) can cause VSMC proliferation and vascular remodeling (4). However, the mechanism has not been fully clarified. Nonetheless, in recent years, it has been found that pyroptosis plays an important role in this process.
Pyroptosis is a type of programmed cell death in which inflammatory cell death is usually caused by microbial infection, stimulation (5). Pyroptosis is often accompanied by inflammasome activation and interleukin-1β (IL-1β) and IL-18 maturation (6). Gasdermin family proteins are the executors of pyroptosis (7). Gasdermin D-N (GSDMD-N) is a member of the gasdermin family. Caspase activation cleaves GSDMD to generate GSDMD-N, and then pores on the cell membrane are formed, the cell permeability is changed, inflammatory factors are released in large quantities, and pyroptosis occurs (8,9). Many studies have indicated that the NOD-like receptor family pyrin domain containing 3 (NLRP3) inflammasome and the induction of pyroptosis play an important role in vascular dysfunction, injury, and remodeling in hypertensive patients (10,11). Tissue factor pathway inhibitor (TFPI) is a plasma Kunitz-type serine protease inhibitor composed of three multivalent Kunitz-type domains produced by microvascular endothelial cells, liver and monocytes, and it modulates the activation of TF-dependent coagulation pathways (12). TFPI participates in the occurrence and development of cardiovascular diseases by inhibiting the proliferation of endothelial cells and VSMCs, reducing thrombin formation, preventing thrombosis, inhibiting the release of inflammatory factors, inducing apoptosis, and blocking matrix metalloproteinases (MMPs) activity (13-15). In addition, many studies have shown that the expression level of TFPI is increased in the plasma of patients with atherosclerotic heart disease and hypertension (16,17).
In our previous study, we found that TFPI expression could inhibit the proliferation of VSMCs; however, whether TFPI can affect hypertensive vascular remodeling by affecting cell pyroptosis has not been reported in the literature (18,19). This study aims to demonstrate that the NLRP3 inflammasome is involved in Ang II-induced VSMC pyroptosis, to verify the effect and possible mechanism of recombinant TFPI (rTFPI) on Ang II-induced VSMC pyroptosis and to provide new ideas for the prevention, occurrence, development, and treatment of hypertensive vascular remodeling.
Methods
Reagents
Anti-GSDMD antibody, anti-pro-caspase-1 + p10 + p12 antibody, and anti-IL-1β antibody for western blotting were all purchased from Abcam (Cambridge, UK). Anti-NLRP3 antibody was purchased from Wanleibio (Shenyang, China). Anti-GSDMD antibody for immunofluorescence was purchased from Bioss (Beijing, China). MCC950 (NLRP3 inhibitor) was purchased from MedChemExpress (Monmouth Junction, NJ, USA). rTFPI was purchased from R&D (Minneapolis, MN, USA).
VSMC culture
Primary VSMCs were isolated from the thoracic aorta of rats using the explantation technique. VSMCs at passages 4–5 were used for the experiments. Sprague-Dawley (SD) rats (100–150 g) were purchased from the Second Affiliate Hospital of Harbin Medical University, Harbin, China. Experiments were performed under a project license (No. 2021117) granted by the institutional ethics board of the First Affiliated Hospital of Harbin Medical University, in compliance with institutional guidelines for the care and use of animals. VSMCs were cultured in a 37 ℃, 5% CO2 incubator with Dulbecco’s modified Eagle medium (DMEM) containing 10% fetal bovine serum (FBS).
Experimental grouping
According to the best values of cell viability as measured by the cell counting kit-8 (CCK8) method, the Ang II concentration used in the experiments was 10−5 mol/L and the rTFPI concentration was 50 µg/L (data not shown). The VSMCs were randomly divided as follows: (I) control group: VSMCs were cultured with DMEM containing 10% FBS; (II) Ang II group: Ang II (10−5 mol/L) was added to the cultured VSMCs; (III) MCC950 group: MCC950 (15 nmol/L) was added into the cultured VSMCs; (IV) Ang II + MCC950 group: Ang II (10−5 mol/L) and MCC950 (15 nmol/L) were added to the cultured VSMCs at the same time; and (V) Ang II + rTFPI group: Ang II (10−5 mol/L) and rTFPI (50 µg/L) were added to the cultured VSMCs at the same time. The cells in each group were starved for 12 hours, treated with drugs, and then cultured in the incubator for 48 hours.
Measurement of cell viability in each group by CCK8 and 3-(4,5-dimethyl-2-thiazolyl)-2,5-diphenyl-2H-tetrazolium bromide (MTT) method
Cell viability in each group was measured by CCK8 assay. VSMCs were placed in 96-well plates in DMEM containing 10% FBS. After incubation in a 37 ℃, 5% CO2 incubator for 12 hours, VSMCs in each group were starved for 12 hours and then underwent different treatments. Forty-eight hours later, 10 µL of CCK8 solution was added to each well, and the absorbance values to determine cell viability were measured at 450 nm with a microplate reader (Hercules, CA, USA).
Cell viability in each group was also measured by MTT assay. Cell processing steps are the same as CCK8 assay. VSMCs were placed in 96-well plates. Added 20 µL of MTT reagent to 200 µL of culture solution per well (protected from light conditions). After placing the 96-well plate in an incubator protected from light for 4 h, all the liquid was discarded, then 150 mL of dimethyl sulfoxide (DMSO) was added, and it was placed on a shaker protected from light for 15 minutes, and measuring the 490 nm absorbance to determine cell proliferation rate.
Propidium iodide (PI) staining and immunofluorescence staining to detect VSMC pyroptosis
The formation of pores on the cell membrane was detected by PI staining. VSMCs were randomly seeded in a 35 mm confocal dish at an appropriate density. After incubation in a 37 ℃, 5% CO2 incubator for 12 hours, VSMCs in each group were starved for 12 hours and then underwent different treatments. PI staining was performed 48 hours later. After fixation with 4% paraformaldehyde, the cells in each group were stained with 4',6-diamidino-2-phenylindole (DAPI). The positive rate of PI staining was calculated under a confocal microscope.
Immunofluorescence staining was also performed to detect VSMC pyroptosis in each group. VSMCs were randomly inoculated into 35 mm confocal dishes at the appropriate density. The drug treatments of the cells were the same as in the PI staining experiment. Immunofluorescence staining was performed 48 hours later. The cells were fixed with 4% paraformaldehyde, incubated in phosphate-buffered saline (PBS) with 0.1% Triton X-100 for 20 minutes at room temperature and blocked with goat serum for 30 minutes at room temperature. Then, the cells were incubated with GSDMD-N antibody (1:100) and Alexa Fluor 594-labeled goat anti-rabbit immunoglobulin G (IgG) (1:100). DAPI was used to counterstain nuclei. The results were observed under a confocal microscope, and the images were collected using ZEN software.
Transmission electron microscopy was performed to observe cell ultrastructural changes and phenotypic transformation
As described previously, cells in each group were fixed in 2.5% glutaraldehyde, postfixed in 1% osmium acid fixation solution after 0.1 M phosphoric acid buffer rinsing, and dehydrated in a series of ethanol. Dehydration was completed in propylene oxide, and the specimens were embedded in embedding liquid. Ultrathin sections were produced on an ultramicrotome, mounted on copper grids, and double-stained with uranium acetate-lead citrate. Specimens were analyzed and documented by electron microscopy (Olympus Companies, Tokyo, Japan).
Western blot analysis
After 48 hours of treatment, cells were washed and resuspended in radioimmunoprecipitation assay (RIPA) buffer lysis buffer [RIPA:phenylmethylsulfonyl fluoride (PMSF) =100:1]. The fully lysed cells were incubated on ice 30–40 minutes, then scrape the cells off and move them to a centrifuge tube, shake them once every 5 minutes, and lyse them on ice for a total of 30 minutes, centrifuge (4 ℃, 13,500 rpm) for 10 minutes and record the volume. The extracted protein was mixed with 5× [sodium dodecyl-sulfate polyacrylamide gel electrophoresis (SDS-PAGE) loading buffer] at a ratio of 4:1 and the protein sample was heated on a heater for 100 ℃ for 10 minutes to denature the protein. The concentration of the extracted protein was measured using a bicinchoninic acid (BCA) protein quantification kit. The protein samples (40 µg) in each group were loaded into the gel and separated by SDS-PAGE. The separated proteins were then transferred onto polyvinylidene fluoride (PVDF) membranes activated by methanol. After blocking with 5% nonfat milk, the membranes were incubated overnight with primary antibodies against NLRP3 (1:2,000), pro-caspase-1 (1:1,000), GSDMD-N (1:1,000), IL-1β (1:2,000), and glyceraldehyde-3-phosphate dehydrogenase (GAPDH) (1:2,000) diluted in blocking buffer. Then, the membranes were washed with tris-buffered saline Tween-20 (TBST) and incubated with horseradish peroxidase-conjugated secondary antibody (1:5,000, Zsbio, Beijing, China). The proteins were detected using the electrogenerated chemiluminescence (ECL) method (Beyotime, Shanghai, China). Images were obtained with a Bio-Rad gel imaging system (Bio-Rad, Hercules, CA, USA). Quantitative analysis of protein bands was performed using ImageJ software.
Statistical analysis
Each experiment was repeated at least four times. The data are shown as the mean ± standard deviation (SD). Experimental data were quantitatively analyzed by GraphPad Prism 8.0 and ImageJ software. The data in each figure achieved normal distribution. One-way analysis of variance (ANOVA) was used for comparisons between multiple groups. A probability value of P<0.05 was considered to be statistically significant.
Results
The viability of VSMCs in each group
To determine whether Ang II could influence VSMC viability and the role of NLRP3 in this process, the CCK8 and MTT methods were used to measure the viability of VSMCs in each group. The results showed that the cell viability in the Ang II group was higher than that in the control group (P<0.05), whereas the cell viability in the Ang II + MCC950 group was significantly lower than that in the Ang II group (P<0.05) (Figures 1,2), which indicated that NLRP3 might participate in the VSMC proliferation induced by Ang II.
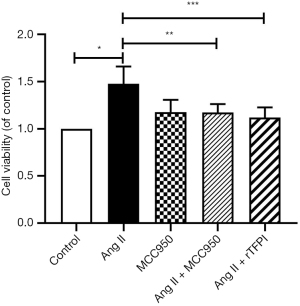
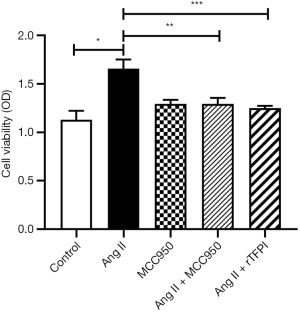
We also investigated whether rTFPI could inhibit Ang II-induced VSMC viability by performing CCK8 and MTT assays. The data showed that the cell viability in the Ang II + rTFPI group was significantly lower than that in the Ang II group (P<0.05) (Figures 1,2). This result suggested that rTFPI might prevent Ang II-induced VSMC proliferation.
PI staining to detect pyroptosis in cells in each group
PI staining, which was used to detect the formation of pores on the cell membrane, was performed to investigate whether Ang II could induce VSMC pyroptosis through the NLRP3 pathway. The results showed that the positive rate of PI staining in the Ang II group was significantly higher than that in the control group (P<0.05, Figure 3), while the positive rate of PI staining in the Ang II + MCC950 group was significantly lower than that in the Ang II group (P<0.05). These results indicated that Ang II might induce pyroptosis through the activation of NLRP3.
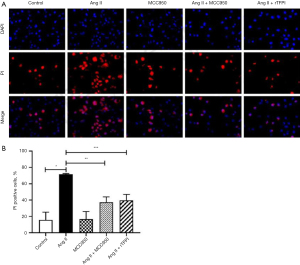
We also verified whether rTFPI could inhibit Ang II-induced VSMC pyroptosis by performing PI staining. The data showed that the positive rate of PI staining in the Ang II + rTFPI group was significantly lower than that in the Ang II group (P<0.05, Figure 3). This suggested that rTFPI could inhibit Ang II-induced VSMC pyroptosis, and we speculated that rTFPI might exert this function by blocking the NLRP3 pathway.
Immunofluorescence staining to detect pyroptosis in cells in each group
To determine whether Ang II could induce VSMC pyroptosis and verify the role of NLRP3 in this process, the expression level of intracellular GSDMD-N, which is a marker protein of pyroptosis, was detected by immunofluorescence staining in each group. The results showed that the expression level of GSDMD-N in the Ang II group was higher than that in the control group (P<0.05, Figure 4), while it was significantly decreased in the Ang II + MCC950 group compared with that in the Ang II group. These results suggested that Ang II might induce the occurrence of pyroptosis through the activation of NLRP3.
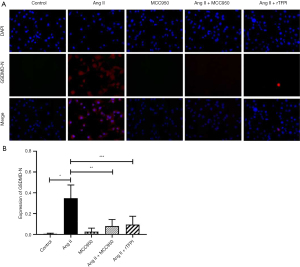
We also determined whether rTFPI could inhibit Ang II-induced expression of GSDMD-N. The data showed that the expression level of GSDMD-N in the Ang II + rTFPI group was significantly lower than that in the Ang II group (P<0.05, Figure 4). This finding suggested that rTFPI could inhibit the expression of GSDMD-N and therefore prevent Ang II-induced VSMC pyroptosis.
VSMC ultrastructural changes and phenotypic transformation observed by transmission electron microscopy
After 48 hours of Ang II induction, the ultrastructural changes and phenotypic transformation of VSMCs in each group were observed with a transmission electron microscope. The results showed that VSMCs in the control group secreted a very small amount of extracellular matrix, the myofilaments were evenly distributed, and the endoplasmic reticulum, Golgi complex, mitochondria, and other organelle structures closely related to protein synthesis were relatively few. Compared with the control group, the VSMCs in the Ang II group secreted a large amount of extracellular matrix, the myofilaments were significantly reduced, and the endoplasmic reticulum, Golgi complex, and mitochondria were significantly increased. Compared with the Ang II group, the VSMCs in the Ang II + rTFPI group secreted less extracellular matrix, the myofilaments were relatively increased, and the endoplasmic reticulum, Golgi complex, and mitochondria were relatively decreased. The above results suggested that after Ang II stimulation of VSMCs, the cell contraction phenotypic characteristics were reduced and the synthetic phenotype features increased. Furthermore, the addition of rTFPI inhibited this phenotype change, proving that rTFPI might inhibit the transformation of Ang II-induced VSMCs from a contractile phenotype to a synthetic phenotype in this process (Figure 5).
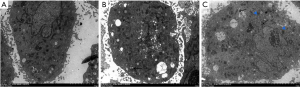
The expression of pyroptosis-related proteins in VSMCs
The expression of pyroptosis-related proteins (such as NLRP3, GSDMD-N, pro-caspase-1, and IL-1β) in VSMCs in each group was detected by western blotting. The results showed that compared with that in the control group, the expression of the pyroptosis-related proteins NLRP3, GSDMD-N, pro-caspase-1, and IL-1β in the Ang II group was significantly increased (P<0.05), whereas the expression of these proteins in the Ang II + MCC950 group was significantly decreased compared with that in the Ang II group (P<0.05, Figure 6). These results indicated that the NLRP3 pathway may be involved in the process of Ang II-induced pyroptosis.
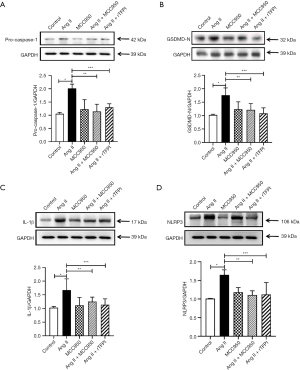
The data also showed that the expression levels of the pyroptosis-related proteins NLRP3, GSDMD-N, pro-caspase-1, and IL-1β were significantly lower in the Ang II + rTFPI group than in the Ang II group (P<0.05, Figure 6). Therefore, we speculated that rTFPI may reduce Ang II-induced pyroptosis by blocking the NLRP3 pathway.
Discussion
RAAS can be involved in the regulation of hypertension through inflammatory and anti-inflammatory pathways (20). One of the pathological foundations of target organ damage in hypertension is hypertensive vascular remodeling; that is, when the internal and external environment of the body changes, the structure and function of the body’s blood vessels undergo adaptive changes. The process by which this change occurs is called vascular remodeling (21). The underlying mechanisms include the inflammatory response, VSMC phenotype transition, endothelial dysfunction, VSMC autophagy, and endoplasmic reticulum stress. When blood vessels encounter stimuli, VSMCs begin to proliferate and migrate by switching phenotypes that alter their gene expression profiles (22). In recent studies, pyroptosis has been increasingly linked to hypertensive vascular remodeling.
Pyroptosis is a novel feature of programmed cell death that occurs during hypertensive VSMCs proliferation. The release of a large number of inflammatory cells leads to cell pyroptosis, further aggravating the release of inflammatory factors. Pyroptosis plays an important role in vascular remodeling in hypertension (23). There are an increasing number of studies on the relationship between pyroptosis and VSMC proliferation and phenotype transition. The release of a large number of inflammatory factors during pyroptosis aggravates the inflammatory response and further aggravates the remodeling of hypertensive blood vessels. By ligating mouse carotid arteries in animal experiments, Wang et al. found that the NLRP3 inhibitor MCC950 could inhibit vascular remodeling, and in cell experiments, MCC950 could inhibit Ang II-induced VSMC proliferation, migration, and phenotypic transformation (24,25). Therefore, the NLRP3 inflammasome is a typical inflammatory factor that induces pyroptosis of VSMCs and vascular remodeling. Although in most cognitions, the cell number decreases after pyroptosis, in this experiment, we found that Ang II-induced pyroptosis in VSMCs and simultaneously increased cell viability to a certain extent. After treatment with MCC950, the cell viability induced by Ang II also decreased. We hypothesized that this might be caused by the stimulatory factors released in the process of pyroptosis in VSMCs, which promote the proliferation of VSMCs. Therefore, we inferred that pyroptosis could promote the proliferation of VSMCs to some extent, and the inhibition of pyroptosis might prevent the proliferation of VSMCs induced by Ang II in hypertension and therefore inhibit vascular remodeling.
The overactivation of Ang II type 1 receptor (AT1-R) by Ang II may be the mechanism underlying the overactivation of the NLRP3 inflammasome, causing VSMC pyroptosis. A large number of studies have found that Ang II-induced inflammation, VSMC proliferation and phenotypic transformation, hypertension, and vascular remodeling can be alleviated by inhibiting NLRP3 gene expression (26). The inflammasome, as a substance that triggers pyroptosis, cleaves GSDMD, forms the GSDMD-N-terminal domain, and finally leads to the formation of a cleavage pore, which is a marker of pyroptosis (9). The inflammasome further amplifies the inflammatory response. The formation of lytic pores leads to changes in cell permeability, and a large number of water molecules then enter the cells, the cells swell, and inflammatory factors are further released (8). Yang et al. applied the NLRP3 inhibitor MCC950 to regulate the inflammatory response, inhibit the infiltration of inflammatory cells, improve the local microenvironment, and further prevent thrombosis and intimal hyperplasia (27).
Although there have been many studies on the proliferation of hypertensive VSMCs, the role of pyroptosis in Ang II-induced VSMC proliferation and vascular remodeling in hypertension is rarely studied. As a new type of programmed cell death, pyroptosis is very different from other modes of cell death; it involves both apoptosis and cell necrosis. Therefore, we used different methods to test the occurrence of pyroptosis in this experiment. The increase in the positive rate of PI staining indicated that the formation of pores on the cell membrane increased. Fluorescence immunostaining revealed that the expression of the pyroptosis-related protein GSDMD-N increased in VSMCs after Ang II treatment, which proved that VSMCs underwent pyroptosis after Ang II treatment. We also found that the protein expression of pyroptosis-related proteins (NLRP3, GSDMD-N, pro-caspase-1, and IL-1β) was significantly increased after Ang II treatment in VSMCs compared with the control group. The above results indicated that Ang II could induce pyroptosis in VSMCs.
Studies on Ang II-induced pyroptosis in VSMCs have not made significant progress in defining the underlying mechanism. Based on previous studies, we treated VSMCs with the NLRP3 inhibitor MCC950 and Ang II at the same time. The results showed that the formation of pores on the cell membrane decreased, immunostaining analysis showed a decrease in GSDMD-N protein expression, and the expression levels of pyroptosis-related proteins (NLRP3, GSDMD-N, pro-caspase-1, and IL-1β) were all decreased. These findings confirmed that inhibiting NLRP3 could reduce Ang II-induced VSMC pyroptosis. These data also suggested that Ang II might induce pyroptosis through the NLRP3 pathway.
A large number of inflammatory factors are released during pyroptosis, and TFPIs can inhibit the inflammatory response by regulating the polarization of macrophages (28). As an endogenous anticoagulant protein, TFPIs play an important role in the blood coagulation pathway, and in vitro studies have confirmed that TFPIs can inhibit the proliferation of vascular smooth muscle (29). Normally, the presence of VSMCs as a systolic phenotype is conducive to fighting vascular tension and maintaining the steady state of the vascular wall. However, when VSMCs are stimulated by certain factors, their phenotype transforms from contractile to synthetic, and they begin to proliferate, migrate, and synthesize too much extracellular matrix, leading to pathological remodeling of blood vessels. In our previous experiments, we found that TFPI could inhibit the occurrence of vascular restenosis by inhibiting VSMC apoptosis and migration, and it could also inhibit the recruitment of inflammatory cells by regulating the upregulation of proinflammatory factors and the downregulation of the expression of anti-inflammatory cytokines (18,30). In this study, electron microscopy results showed that rTFPI could significantly suppress the changes in the VSMC ultrastructure caused by Ang II compared with the Ang II alone group. By microscopy analysis, we found that compared with the Ang II group, there was a relatively decreased secretion of extracellular matrix, a relative increase in muscle filaments, and a relatively decreased endoplasmic reticulum, Golgi complex, and mitochondria in the Ang II + rTFPI group. These findings suggested that rTFPI may inhibit the cell phenotype switch induced by Ang II.
At present, there are no related studies on TFPI and pyroptosis. Since inflammation is one of the factors that induce pyroptosis, we asked whether TFPI could regulate pyroptosis by regulating the inflammatory response. Therefore, in our study, we treated cells with rTFPI to detect Ang II-induced VSMC proliferation and pyroptosis and found that rTFPI not only significantly inhibited Ang II-induced VSMC proliferation but also decreased the positive rate of PI staining and the expression level of GSDMD-N. The expression level of GSDMD-N in the Ang II + rTFPI group was significantly lower than that in the Ang II group, as shown by immunofluorescence. In addition, western blot results showed that rTFPI could reduce the expression of pyroptosis-related proteins, decrease the release of inflammatory factors, and inhibit the release of the NLRP3 inflammasome. Therefore, we speculated that rTFPI might inhibit Ang II-induced VSMC pyroptosis by inhibiting NLRP3 inflammasome expression, thereby interfering with vascular remodeling due to VSMC proliferation in hypertension.
Conclusions
In conclusion, our findings demonstrated that Ang II could promote pyroptosis in VSMCs by activating the NLRP3 inflammasome. Furthermore, rTFPI could inhibit Ang II-induced VSMC pyroptosis, and we found that it may play a role in this process by inhibiting the NLRP3 inflammasome. By exploring the effect of TFPI on vascular smooth muscle and its possible mechanism, our research may provide a new direction for the prevention and treatment of vascular remodeling in hypertension in the future.
Acknowledgments
Funding: This study was supported by the National Natural Science Foundation of China (Nos. 82170513 and 81200143), the Heilongjiang Traditional Chinese Medicine Scientific Research Project (No. ZHY12-W035), and the Foundation of the First Affiliated Hospital of Harbin Medical University (No. 2015B002).
Footnote
Data Sharing Statement: Available at https://cdt.amegroups.com/article/view/10.21037/cdt-23-355/dss
Peer Review File: Available at https://cdt.amegroups.com/article/view/10.21037/cdt-23-355/prf
Conflicts of Interest: All authors have completed the ICMJE uniform disclosure form (available at https://cdt.amegroups.com/article/view/10.21037/cdt-23-355/coif). All authors report that the study was supported by the National Natural Science Foundation of China (Nos. 82170513 and 81200143), the Heilongjiang Traditional Chinese Medicine Scientific Research Project (No. ZHY12-W035), and the Foundation of the First Affiliated Hospital of Harbin Medical University (No. 2015B002). The authors have no other conflicts of interest to declare.
Ethical Statement: The authors are accountable for all aspects of the work in ensuring that questions related to the accuracy or integrity of any part of the work are appropriately investigated and resolved. Experiments were performed under a project license (No. 2021117) granted by the institutional ethics board of the First Affiliated Hospital of Harbin Medical University, in compliance with institutional guidelines for the care and use of animals.
Open Access Statement: This is an Open Access article distributed in accordance with the Creative Commons Attribution-NonCommercial-NoDerivs 4.0 International License (CC BY-NC-ND 4.0), which permits the non-commercial replication and distribution of the article with the strict proviso that no changes or edits are made and the original work is properly cited (including links to both the formal publication through the relevant DOI and the license). See: https://creativecommons.org/licenses/by-nc-nd/4.0/.
References
- Te Riet L, van Esch JH, Roks AJ, et al. Hypertension: renin-angiotensin-aldosterone system alterations. Circ Res 2015;116:960-75. [Crossref] [PubMed]
- Pacurari M, Kafoury R, Tchounwou PB, et al. The Renin-Angiotensin-aldosterone system in vascular inflammation and remodeling. Int J Inflam 2014;2014:689360. [Crossref] [PubMed]
- Tang HY, Chen AQ, Zhang H, et al. Vascular Smooth Muscle Cells Phenotypic Switching in Cardiovascular Diseases. Cells 2022;11:4060. [Crossref] [PubMed]
- Cau SB, Bruder-Nascimento A, Silva MB, et al. Angiotensin-II activates vascular inflammasome and induces vascular damage. Vascul Pharmacol 2021;139:106881. [Crossref] [PubMed]
- Yu P, Zhang X, Liu N, et al. Pyroptosis: mechanisms and diseases. Signal Transduct Target Ther 2021;6:128. [Crossref] [PubMed]
- Ren H, Yang H, Yang X, et al. Brucella Outer Membrane Lipoproteins 19 and 16 Differentially Induce Interleukin-18 Response or Pyroptosis in Human Monocytic Cells. J Infect Dis 2021;224:2148-59. [Crossref] [PubMed]
- Shao F. Gasdermins: making pores for pyroptosis. Nat Rev Immunol 2021;21:620-1. [Crossref] [PubMed]
- Tan Y, Chen Q, Li X, et al. Pyroptosis: a new paradigm of cell death for fighting against cancer. J Exp Clin Cancer Res 2021;40:153. [Crossref] [PubMed]
- Xia S, Zhang Z, Magupalli VG, et al. Gasdermin D pore structure reveals preferential release of mature interleukin-1. Nature 2021;593:607-11. [Crossref] [PubMed]
- Xu S, Chen H, Ni H, et al. Targeting HDAC6 attenuates nicotine-induced macrophage pyroptosis via NF-κB/NLRP3 pathway. Atherosclerosis 2021;317:1-9. [Crossref] [PubMed]
- Sundaram B, Kanneganti TD. Advances in Understanding Activation and Function of the NLRC4 Inflammasome. Int J Mol Sci 2021;22:1048. [Crossref] [PubMed]
- Mast AE. Tissue Factor Pathway Inhibitor: Multiple Anticoagulant Activities for a Single Protein. Arterioscler Thromb Vasc Biol 2016;36:9-14. [Crossref] [PubMed]
- Hong J, Liu R, Chen L, et al. Conditional knockout of tissue factor pathway inhibitor 2 in vascular endothelial cells accelerates atherosclerotic plaque development in mice. Thromb Res 2016;137:148-56. [Crossref] [PubMed]
- Zhao Y, Zhu M, Chen W, et al. TFPIalpha alleviated vascular endothelial cell injury by inhibiting autophagy and the class III PI3K/Beclin-1 pathway. Thromb Res. 2020;195:151-7. [Crossref] [PubMed]
- Zhao Y, Chen W, Liu Y, et al. Promoting plaque stability by gene silencing of monocyte chemotactic protein-3 or overexpression of tissue factor pathway inhibitor in ApoE-/- mice. J Drug Target 2021;29:669-75. [Crossref] [PubMed]
- Zhao Y, Yu Y, Shi M, et al. Association study to evaluate TFPI gene in CAD in Han Chinese. BMC Cardiovasc Disord 2017;17:188. [Crossref] [PubMed]
- Kobayashi H, Matsubara S, Yoshimoto C, et al. Tissue Factor Pathway Inhibitors as Potential Targets for Understanding the Pathophysiology of Preeclampsia. Biomedicines 2023;11:1237. [Crossref] [PubMed]
- Fu Y, Zhao Y, Liu Y, et al. Adenovirus-mediated tissue factor pathway inhibitor gene transfer induces apoptosis by blocking the phosphorylation of JAK-2/STAT-3 pathway in vascular smooth muscle cells. Cell Signal 2012;24:1909-17. [Crossref] [PubMed]
- Fu Y, Ma D, Liu Y, et al. Tissue factor pathway inhibitor gene transfer prevents vascular smooth muscle cell proliferation by interfering with the MCP-3/CCR2 pathway. Lab Invest 2015;95:1246-57. [Crossref] [PubMed]
- Drummond GR, Vinh A, Guzik TJ, et al. Immune mechanisms of hypertension. Nat Rev Immunol 2019;19:517-32. [Crossref] [PubMed]
- Shi N, Mei X, Chen SY. Smooth Muscle Cells in Vascular Remodeling. Arterioscler Thromb Vasc Biol 2019;39:e247-52. [Crossref] [PubMed]
- Zou F, Li Y, Zhang S, et al. DP1 (Prostaglandin D(2) Receptor 1) Activation Protects Against Vascular Remodeling and Vascular Smooth Muscle Cell Transition to Myofibroblasts in Angiotensin II-Induced Hypertension in Mice. Hypertension 2022;79:1203-15. [Crossref] [PubMed]
- De Miguel C, Pelegrín P, Baroja-Mazo A, et al. Emerging Role of the Inflammasome and Pyroptosis in Hypertension. Int J Mol Sci 2021;22:1064. [Crossref] [PubMed]
- Wang GQ. The role of NLRP3 inhibitor MCC950 in vascular remodeling. Qingdao: Qingdao University; 2021.
- Hong P, Li FX, Gu RN, et al. Inhibition of NLRP3 Inflammasome Ameliorates Cerebral Ischemia-Reperfusion Injury in Diabetic Mice. Neural Plast 2018;2018:9163521. [Crossref] [PubMed]
- Ward R, Li W, Abdul Y, et al. NLRP3 inflammasome inhibition with MCC950 improves diabetes-mediated cognitive impairment and vasoneuronal remodeling after ischemia. Pharmacol Res 2019;142:237-50. [Crossref] [PubMed]
- Yang GY. Embryonic stem cell-derived endothelial cells combined with MCC950 to construct functional tissue-engineered blood vessels. Chongqing: PLA Army Medical University; 2020.
- Espada S, Stavik B, Holm S, et al. Tissue factor pathway inhibitor attenuates ER stress-induced inflammation in human M2-polarized macrophages. Biochem Biophys Res Commun 2017;491:442-8. [Crossref] [PubMed]
- Han X, Girard TJ, Baum P, et al. Structural requirements for TFPI-mediated inhibition of neointimal thickening after balloon injury in the rat. Arterioscler Thromb Vasc Biol 1999;19:2563-7. [Crossref] [PubMed]
- Yan R, Gao W, Chen W, et al. rTFPI Protects Cardiomyocytes from Hypoxia/Reoxygenation Injury through Inhibiting Autophagy and the Class III PI3K/Beclin-1 Pathway. Cell Biochem Biophys 2023;81:97-104. [Crossref] [PubMed]